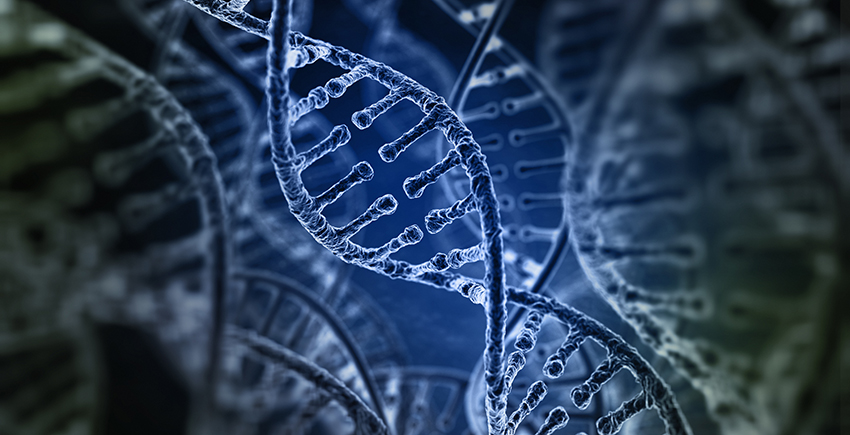
This article is part of Harvard Medical School’s continuing coverage of medicine, biomedical research, medical education and policy related to the SARS-CoV-2 pandemic and the disease COVID-19.
Why do some people fall ill from SARS-CoV-2 while others infected with the virus experience no symptoms?
Why do some people develop neurological, cardiovascular or gastrointestinal symptoms while infection sticks to the respiratory system in others?
Since older age and underlying health conditions put people at greater risk of becoming seriously sick or dying from COVID-19, why do some young and otherwise healthy people barely survive the disease, while some elderly people with multiple risk factors weather it with only minor symptoms?
In addition to individual behavior, preexisting conditions and sheer luck, answers could lie in people's genes.
"We have an opportunity to see if there are gene variants that distinguish people who are more susceptible to the virus from those who are more resistant," said Ting Wu, professor of genetics at Harvard Medical School.
Geneticists, including many in the Blavatnik Institute at HMS, have joined scientists across disciplines to investigate critical questions like these as the COVID-19 pandemic engulfs the globe, infecting millions and killing hundreds of thousands.
They work day and night to understand the new coronavirus in the hope that their insights will help fellow researchers identify or design effective treatments, develop vaccines, track infections and prevent future illness and death.
While most on-campus research at HMS ramped down in March to safeguard community health, a handful of labs in the Department of Genetics secured permission to continue limited in-person operations for projects that could have immediate impact on the COVID-19 pandemic. Others have found ways to contribute from home.
"We're all trying to throw our expertise at this," said Jenny Yan, a biological and biomedical sciences PhD student at HMS specializing in genetics and genomics.
While some HMS geneticists scrutinize the human genome, others are analyzing the genome of the virus to track the spread of different lineages around the world and help determine whether any mutations affect the virus's potency.
Some are studying gene dynamics in cell cultures and animal models to uncover clues about how the body responds to the virus at the molecular level, whether that's granting entry to SARS-CoV-2 or sparking a cascade that leads to tissue and organ damage or runaway inflammation.
Still others are applying their knowledge of DNA- and RNA-based technologies to improve existing tests and develop new ones that can diagnose COVID-19 or detect evidence of past infection.
It's not clear which of these experiments will yield useful information, nor how quickly. In science, after all, failures outnumber successes, and discovery takes time.
What keeps the researchers going is the possibility of making discoveries or developing tools that identify weaknesses in the virus or strengths in the human body, inform public policy, protect human health today or help prevent future pandemics.
"The virus has caused so much suffering and economic downturn, we should shoot everything at it that we can," said Harald Ringbauer, HMS postdoctoral research fellow in genetics. "It's important right now to learn more about the virus and its behavior, and genetics has a huge potential to teach us these things."
In search of genetic outliers
Getting a complete picture of which genes, if any, affect human susceptibility to the new coronavirus will take a long time and the analysis of many thousands of people's genomes. In the meantime, Wu and colleagues are getting a jump start by using an existing resource, the Personal Genome Project (PGP), and by looking at the edge cases, the extremes.
"We're fishing for genetic outliers—those who are way out on the ends of the COVID-19 bell curve," said George Church, the Robert Winthrop Professor of Genetics at HMS and a founding core faculty member of the Wyss Institute for Biologically Inspired Engineering, whose lab is pursuing multiple endeavors related to the COVID-19 pandemic.
"We don't necessarily need to do a deep dive on 7 billion people to help us predict who's likelier to get sick or who's asymptomatic but shouldn't be exposed to other people because they're still shedding virus," he said.
Launched in 2005 in the Church lab and now spanning multiple countries, the PGP invites volunteers to share their health and genetic information for scientific research. The program currently includes more than 6,000 participants, about 500 of whom have uploaded their complete genome sequences. Church is one of them.
The team plans to fish for clues in self-reported data collected from participants, such as ZIP code, gender, race and ethnicity, health conditions and smoking and vaping behavior as well as details about any COVID-19 symptoms, test results and potential exposures to the virus. If individual participants stand out, the team can analyze any DNA they've submitted.
Wu also envisions recruiting more participants in COVID-19 hotspots to help uncover relevant gene variants, using survey results to prioritize participants for gene sequencing.
If the PGP team or other researchers do find variants that raise or lower risk of serious illness or death from COVID-19, it's possible that people could then be tested for those variants. That could in turn make it easier to determine who can most safely work on the front lines in certain industries and who should be more protected, said Wu, as well as who should take extra precautions in communities, such as people found to be at high risk of carrying and spreading the virus without experiencing symptoms themselves.
The PGP offers only one of many ways to probe the genetics of SARS-CoV-2 infection. It has its weaknesses—for one, the repository is smaller than other international genetic databases—but it has already received IRB approval and boasts an eager patient base.
"PGP is unique in its readiness," said Wu, who is also an associate faculty member of the Wyss. "This round may or may not generate any useful results, but it shows how well an existing program can be prepared to act on emerging needs—and how planning ahead can be helpful."
"With enough attention and funding, programs like this can be poised to respond to the next pandemic," she added.
Project team members are Sarah Wait Zaranek, Alexander Wait Zaranek, Ranjan Ahuja, Michael Chou, Jason Bobe, Preston Estep and Jeantine Lunshof.
Insights from ancient-DNA research
What does the migration of groups of early humans out of Africa have to do with the spread of SARS-CoV-2 in 2020? Similar genome-analysis techniques are illuminating both.
As SARS-CoV-2 infects people and replicates, the individual viruses evolve, acquiring genetic mutations. Different sets of mutations begin to distinguish different lineages of the virus, which can be linked to different parts of the world. Tracking these mutations allows researchers to study how the virus is moving around.
Viruses sampled from a cluster of patients in Baton Rouge, for example, might share a unique genetic fingerprint, indicating local spread of SARS-CoV-2, while a patient in New York City might harbor a version of the virus almost identical to one circulating in Italy, suggesting that human travel contributed to infection.
The genetic sequencing and computational techniques used to follow the movement of SARS-CoV-2 lineages and tie them to specific locations resemble those used to reconstruct how groups of people tens of thousands of years ago were related to one another and how they moved around the globe based on DNA recovered from ancient human bones. That's no coincidence: Many of the tools used in ancient-DNA research were first developed for virology.
HMS geneticists accustomed to probing prehistory are now turning their talents to COVID-19.
"We're hoping to reinfuse into virology the expertise we've gained from studying ancient DNA," said postdoc Ringbauer, who is based in the lab of ancient-DNA specialist David Reich, professor of genetics at HMS. Reich also holds appointments at Harvard University and the Broad Institute of MIT and Harvard.
"There are already, of course, great scientists working on the new coronavirus," said Ringbauer. "We just hope we can contribute a little."
Ancient-DNA researchers have several skills to offer, such as familiarity with analyzing big data sets. When scientists studied Zika and Ebola virus strains in the last decade, they worked with a few hundred sequences, said Ringbauer. As of June 10, more than 42,000 genome sequences have been collected for SARS-CoV-2.
The virus replicates by cloning its RNA, creating identical copies of itself (except for any new mutations). Most human DNA, by contrast, replicates through recombination, the mixing of genetic material from two parents.
But ancient-DNA researchers also specialize in studying the two exceptions to this rule: Y chromosomes, which are passed from male parent to male offspring without recombining, and mitochondrial DNA, which is inherited wholesale from the mother.
Tracking similarities and differences in Y-chromosome and mitochondrial DNA, which evolve like viral RNA, have made it possible to narrow down the timing of out-of-Africa and other migrations and reconstruct maternal and paternal family trees across millennia, just as tracking viral RNA from patient to patient can reconstruct the SARS-CoV-2 family tree in real time.
When they've finished collecting and cleaning up the data, Ringbauer and Reich will analyze SARS-CoV-2 genomes with the goal of helping researchers answer many questions about the pandemic, including: How mobile is the virus? How many flames is it fanning with sparks that originated locally versus far away? Are most cases caused by a few super-spreaders, or do most people have a few interactions on average with those who've been infected?
And do any of the mutations change the virus's behavior, making it easier or harder to transmit or more or less likely to cause disease—essentially creating a new strain?
With answers that could inform public policy, the project was one of 62 proposals awarded funding in a first round of grants from the Massachusetts Consortium on Pathogen Readiness (MassCPR).
Fly fishing
Viruses can't infect people unless some of our cells let them in. Certain proteins on cell surfaces grant entry to SARS-CoV-2, while others inside the cell get co-opted to help the virus replicate.
By uncovering each of the proteins at play, scientists provide new opportunities to find drugs that act on the proteins and make it harder for the virus to gain a foothold.
Researchers have identified major culprits, such as the ACE2 receptor and molecular scissors known as TMPRSS2, that roll out a welcome mat for the new coronavirus. Three HMS geneticists contributed to an international study in Nature Medicine in April detailing the activity of genes that make these proteins in cells lining the airways, blood vessels, heart, cornea and intestine.
The contributors were Deborah Hung, HMS professor of genetics at Massachusetts General Hospital; Christine Seidman, the Thomas W. Smith Professor of Medicine at HMS and Brigham and Women’s Hospital; and Jonathan Seidman, the Henrietta B. and Frederick H. Bugher Foundation Professor of Genetics at HMS.
But the list of genes and proteins may not yet be complete. Norbert Perrimon, the James Stillman Professor of Developmental Biology at HMS, hopes to add to it using genetic screens of fruit fly cells.
Why fly cells? For one thing, human and other mammalian genomes are complex; a single gene can make more than one protein, and a single protein can be made by more than one gene. Fly genomes are simpler, making it easier to flag the true actors involved in viral entry.
"We might identify proteins in the fly that have equivalents in humans but that would have been missed from mammalian studies," said Perrimon.
Many, but not all, basic biologic mechanisms discovered in flies also hold true for other organisms, including people. If Perrimon's team can generate a list of genes and proteins that seem to be related to SARS-CoV-2 entry in fly cells, researchers can compare it to lists generated from human and mammalian studies and use the overlaps to home in on the factors most likely to prove important.
The team needs to clear a few hurdles first, though. For instance, flies in the wild don't get COVID-19, so Perrimon and research fellow in genetics Raghuvir (Ram) Viswanatha must find out whether fly cells let in SARS-CoV-2 particles naturally. If not, the researchers will engineer the cells to express human ACE2 and TMPRSS2, and if that does the trick, they can then watch which additional genes kick into gear as the virus makes its way inside.
The idea isn't so farfetched. Flies don't get the flu, either, but the same technique has allowed scientists to use the insects to learn about how the influenza virus enters cells.
"If it works, we should get some interesting results," said Perrimon. "If not, well, that's the end of the project."
If the fly cells do grant entry to SARS-CoV-2 one way or the other, Viswanatha will use a platform he developed based on the gene editing tool CRISPR to swiftly identify all the genes that appear to be involved.
Viswanatha was using the platform earlier this year to uncover proteins that fly cells use to let in an insect pathogen when scientists announced that some of the same proteins might be involved in SARS-CoV-2 infection.
Although the researchers aren't sure their experiment will pan out, they have enough confidence to believe it's worth a try.
"If we could find new factors involved in the entry of the virus that lead to a complete 'parts list,' that would be a good achievement," said Perrimon. "We would be happy."
Chasing an RNA tail
When Jenny Yan and colleagues began studying RNA—molecules that translate DNA’s instructions into proteins—in Caenorhabditis elegans worms, she didn't expect that the team's findings might become relevant to a global pandemic.
"We're a very basic science lab," said Yan, a research assistant in the lab of Scott Kennedy, the Philip and Aya Leder Professor of Genetics at HMS. "We're interested in things like gene expression and worm biology."
Many RNAs end in a string of repeating adenine bases: a so-called poly(A) tail. In May in the journal Nature, Kennedy's group reported that they found a previously unknown type of RNA with a repeating uracil and guanine tail in C. elegans. The researchers dubbed them poly(UG) or pUG RNAs.
Both pUG and poly(A) tails allow RNAs to recruit other proteins that perform important jobs, such as RNA polymerase, which makes copies of RNA molecules.
The week the researchers posted their manuscript on a preprint server in Dec. 2019, the first case of what is now known as COVID-19 was reported in China. It soon became clear that SARS-CoV-2 belongs to a family of viruses with genomes made of RNA rather than DNA.
Countless groups jumped to apply knowledge of RNA to combat the new threat. Kennedy wondered if his team could contribute.
A few researchers in the lab are now trying to find out: Does the coronavirus contain pUG RNAs? If so, what does it use them for? And can they then become a treatment target?
The team has detected a few promising signs so far, but the work has only just begun, and it's not yet clear whether the initial signals are real.
"It's enough to be motivating, but we're not convinced yet," said Yan. "It's still very preliminary. We have some hints we need to verify."
If SARS-CoV-2 does use pUG RNAs to recruit RNA polymerase, scientists could then try to disrupt the signals that attach the tail or recruit the enzyme so the virus can't replicate.
If the virus contains pUG RNAs but they don't behave the same way as in worms, Kennedy and colleagues have a few other guesses as to what the molecules might be doing and how those might suggest antiviral strategies.
And if the researchers don't detect any pUG RNAs, the lab will likely return its full attention to the work it was pursuing pre-pandemic—unless new inspiration strikes.
"That's the beautiful thing about research; you don't know where a project will take you," said Yan.
Testing, testing
Testing for current or past SARS-CoV-2 infection is a cornerstone of understanding and controlling the spread of COVID-19, but testing capacity and reliability in the U.S. have not yet met the nation's needs. Researchers are trying to change that.
The lab of Stephen Elledge, the Gregor Mendel Professor of Genetics and of Medicine at HMS and Brigham and Women’s, has updated its comprehensive antibody-detection tool, VirScan, to flag past exposure to SARS-CoV-2 in blood samples and help immunologists and epidemiologists better gauge the virus's lethality, population exposure rates, and short- and long-term effects on the immune system. Elledge received MassCPR funding for these efforts.
The Church lab has joined nationwide efforts to build faster, cheaper, more accessible tests to diagnose COVID-19. So have the labs of Connie Cepko, the Bullard Professor of Genetics and Neuroscience at HMS, and Steven McCarroll, the Dorothy and Milton Flier Professor of Biomedical Science and Genetics at HMS.
Brian Rabe, a PhD candidate in biological and biomedical sciences in the Cepko lab, is adapting an existing assay to detect SARS-CoV-2 in patient samples in half an hour and without the need for specialized equipment or training. The work builds on Cepko's expertise in using viruses as research tools.
Rabe and colleagues are working on improvements such as stabilizing and deactivating viral particles in patient samples. If they can make the necessary changes, the researchers hope the test could eventually be deployed in doctor's offices and other health care facilities.
Meanwhile, research assistant in genetics Curtis Mello and other members of McCarroll's team have focused on a different technology—nanoliter droplets combined with digital PCR—to more precisely measure and characterize viral RNA in patient samples and thus provide more reliable COVID-19 diagnoses.
The method is designed to distinguish large viral RNA molecules, which are produced by live, replicating viruses, from fragmented viral RNA, which may be shed long after an active infection.
Current tests, by contrast, typically deliver a positive result if they detect any viral RNA, whether whole or broken. This could explain at least some of the cases where people test positive for COVID-19 even though their symptoms have faded, the researchers said.
In McCarroll's technique, individual patient samples get enclosed in microscopic droplets along with chemicals required for amplifying and detecting genetic material from the coronavirus. Tens of thousands of these droplets can be analyzed simultaneously.
Droplets are engineered to glow if they contain SARS-CoV-2 RNA. Those that contain long, intact pieces of RNA glow in multiple colors. Glowing droplets get counted digitally as they flow through a microfluidic device. Digital counting should provide more accurate results than traditional PCR (polymerase chain reaction), the standard method for amplifying genetic material, the researchers said.
The goals are to assist epidemiologists, help measure infectiousness at different points throughout illness and quickly detect active virus particles on surfaces so people can keep spaces safe for living and working.
Additional lab members working on the project are Nolan Kamitaki, research associate in genetics, and Heather de Rivera, researcher in natural science. The research is supported by the HMS Department of Genetics.
Images, from top: iStock, N/A, © Martin Frouz and Jiří Svoboda, Perrimon lab, Kennedy lab, Pond5