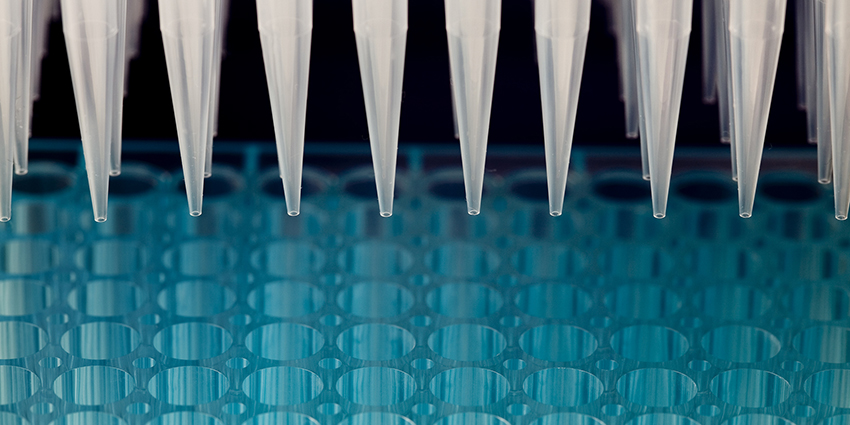
This article is part of Harvard Medical School’s continuing coverage of medicine, biomedical research, medical education and policy related to the SARS-CoV-2 pandemic and the disease COVID-19.
The COVID-19 pandemic demands action on many fronts, from prevention to testing to treatment.
Not content to focus its research efforts on just one, the laboratory of George Church in the Blavatnik Institute at Harvard Medical School and the Wyss Institute for Biologically Inspired Engineering at Harvard University is tackling the problem from seven different angles.
Partnering with various colleagues, the team's goals are to:
- Create simple, cheap, more accessible testing for SARS-CoV-2, the virus that causes COVID-19, both in humans and on various surfaces.
- Develop a faster way to identify antibodies that neutralize the virus.
- Generate lung tissue models called organoids to test candidate drugs for COVID-19.
- Harness the power of individual genomes to identify variants that raise or lower people's risk of serious illness when infected by SARS-CoV-2.
- Increase mask use in public.
- Develop public health strategies.
- Identify any antibodies that inadvertently help the virus enter cells so researchers who are developing vaccines can avoid including those antibodies.
The projects "get at different parts of the elephant," said Church, the Robert Winthrop Professor of Genetics at HMS, comparing the many facets of the pandemic to the parable about several blind men who are each touching different parts of the animal and unable to characterize it as a whole.
"It's too late for certain types of prevention" to contain the spread of the new coronavirus, he said, but there are opportunities to pursue other interventions in the short- and long-term that could save lives.
Most HMS labs have transitioned to remote work following institutional guidance intended to contain the spread of the virus, but some have been granted permission to continue on-site work for COVID-19-related projects. A small fraction of the Church lab is among them.
"We, like some other research labs, already had projects that were adjacent to COVID-19, so it was not hard for us to pivot a little bit," said Church, who is a founding core faculty member of the Wyss Institute.
Church and Ting Wu, professor of genetics at HMS, associate faculty member at the Wyss Institute and principal investigator of the genomics-focused project, have applied for funding through the HMS-led Massachusetts Consortium on Pathogen Readiness, or MassCPR. Although the winning applications have not yet been announced, Church said he will find a way to pursue the work regardless.
Three of the projects are described below.
Building better tests
It's become clear that there aren't enough tests in the U.S. to identify everyone who is sick with COVID-19, let alone to detect people who are infected with the new coronavirus but experiencing mild or no symptoms.
The lack of population-wide testing hampers efforts to accurately assess—and stop—the spread of SARS-CoV-2.
"A number of us are convinced that the big difference between the places that are doing well and the ones that aren't are high levels of testing," said Church.
Many groups are striving to overcome testing bottlenecks by ramping up test production and processing and by investigating ways to improve the efficiency and accuracy of the tests themselves. The Church lab has joined their efforts.
The lab is exploring two paths. First, the researchers are trying to develop a tool that can process up to 1 million samples per day. That would be a vast improvement over current testing capacity, estimated to be 160,000 samples per day in the entire U.S. as of April 15, according to The COVID Tracking Project—not enough to cover even those who are symptomatic.
Right now, nasal and throat swabs are sent to labs, where technicians determine whether a given sample contains the new coronavirus by isolating any viral RNA, turning it into DNA and then using a test known as PCR (polymerase chain reaction) to amplify the DNA to detectable levels. The method has several drawbacks, including the limited number of testing slots, or wells, on a PCR plate—typically 96 or 384—and the time and money it takes to synthesize the millions of unique DNA snippets called primers needed to amplify the virus' genetic material.
Church and colleagues intend to bypass these hurdles by giving each anonymized patient sample a unique genetic bar code; mixing hundreds of thousands of samples together; and amplifying the viral RNA in them all at once using a simpler alternative to PCR known as isothermal amplification, which isn't limited by number of wells. When the results are spit out in bulk at the end, the bar codes make it clear who is positive or negative for the virus. The method requires only 72 primers, saving additional time and money.
The team would then partner with clinicians and diagnostic laboratories to make sure people can operate the tests in real-world settings and that the devices meet FDA requirements.
It's not yet clear whether samples would be collected only in health care settings or whether people could receive collection kits at home and mail them to central testing facilities.

Alternatively, Church is investigating the possibility of mass-producing individual test kits that could be fully completed at home, "like a pregnancy test," he said, so that infectious material wouldn't need to be sent through the mail.
Tests also could help workers detect the virus on surfaces in hospitals or other facilities, Church said.
Whether centralized or distributed, high-throughput rapid testing is key to getting ahead of the next wave of infections, he said.
Lab members working on this project are Gabe Filsinger, Raphael Ferreira, Dima Ter-Ovanesyan, Lindsey Robinson-McCarthy, Alex Mijalis, Jenny Tam and Oliver Dodd.
On the hunt for antibodies
About five to ten days after being infected with SARS-CoV-2, the body's immune system generates an array of antibodies, some of which can disable, or neutralize, the virus. Researchers want to identify those antibodies so they can use them to develop treatments, as has been done for viruses such as HIV and West Nile. But that method typically takes too long to be useful against an emerging pathogen, said Church.
One shortcut, currently under investigation for COVID-19 treatment, is to collect antibody-rich plasma from people who've recovered from the disease and transfuse it into patients struggling to fight off infection. Although that strategy has treated other diseases in the past, it has not yet been proven to work for COVID-19, must be performed under carefully controlled circumstances, and doesn't reveal which antibodies in the plasma, if any, are effective.
As an alternative to plasma therapy, Church wants antibody identification and treatment to be a relevant option in the short term. So his group is seeking to build a platform that shrinks the timeline from months to days.
The plan:
- Use computers to design antibodies that are likely to bind to SARS-CoV-2 and therefore have a chance of neutralizing it.
- Build DNA libraries to synthesize the proteins, generating millions of candidate antibodies.
- Screen the antibody candidates in lab dishes to find the ones that tightly bind to SARS-CoV-2.
- Genetically iterate on the best candidates until they bind even more effectively.
- In partnership with the Draper labs at MIT, use single-cell microfluidic devices to sift through about 100 antibody candidates per day and flag those that best neutralize the virus in human cells.
- Share the top binders with other researchers for testing in animal models.
"Neutralizing antibodies are a quick way of dealing with almost any new virus," said Church. "It doesn't require a brand-new category of drugs."
Lab members working on this project are Kettner Griswold, Nick Barry, Per Greisen, David Thompson, Isaac Han, Sergey Ovchinnikov, Samaneh Mesbahi-Vasey and Richie Kohman.
Lungs in the lab
A staggering number of drug candidates fail when they reach human clinical trials because they prove either unsafe or ineffective. These failures stem from significant differences between cells in a lab dish, animal models and the human body.
To circumvent this challenge for COVID-19 therapeutics, the Church lab plans to build a testing platform that mimics human lungs more closely than typical models.
The team intends to start by rapidly producing massive quantities of lung stem cells using a tool called TFome that the Church lab built a few years ago. TFome contains the largest known library of human transcription factors: proteins that convert DNA into RNA and help regulate gene expression. Using TFome to identify and deploy specific combinations of transcription factors, scientists can quickly and efficiently reprogram induced pluripotent stem cells into the specialized cells they want.
In this case, those are lung cells called type II pneumocytes. Found in alveoli, the lung structures where carbon dioxide is exchanged for oxygen, type II cells have two major jobs: releasing filmy surfactant to aid in gas exchange and generating new type I alveolar cells if those critical cells are damaged by toxins or conditions such as acute respiratory distress syndrome—one of the most serious complications of COVID-19, which often requires putting patients on ventilators.
Church anticipates that the TFome method will allow his team to churn out 1 billion type II lung cells in less than four days.
The researchers will test how well the cells embody the most relevant characteristics of normal type II pneumocytes: production of surfactant and the proteins that SARS-CoV-2 uses to enter and infect cells, particularly ACE2 and TMPRSS2.
If they can create a batch of faithfully replicated type II cells, the team will incorporate them into existing lung organoids—3D tissue structures that act like simplistic organs, a middle ground between cell cultures in a lab dish and actual human lungs. Adding type II pneumocytes into the mix of type I pneumocytes and immune cells will bring the organoids physiologically closer to real lungs.
"You want to be able to simulate these cells in their right ratio and ideally their correct geometry, and with the right density of receptors," said Church.
The researchers then want to kick off rapid, large-scale production of the upgraded lung organoids so they can be distributed to labs around the world for drug testing.
TFome-powered organoid production should be faster, more efficient and of more consistent quality than other stem cell-generating protocols and lung-on-a-chip models, Church said.
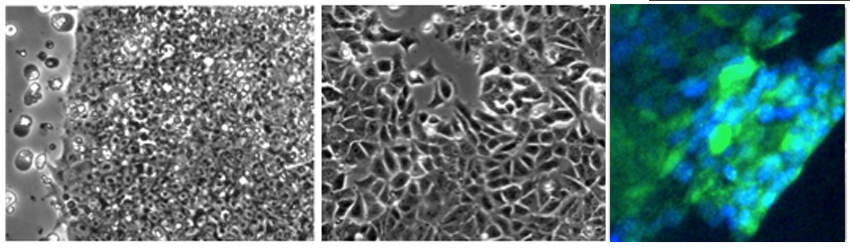
The lung stem cells and organoids could also aid in the study of how infection with SARS-CoV-2 leads to disease. Church would like to catalog gene mutations in the virus' spike protein to assess the infectivity and other characteristics of new substrains of the virus. The results would inform the development of treatments and, perhaps more importantly, vaccines that guard against several strains of the virus.
Sharing any results as quickly as possible with other researchers will help the world prepare against this and future outbreaks, he said.
Lab members working on this project are Parastoo Khoshakhlagh, Alex Ng, Khaled Said and Kiavash Kiaee.