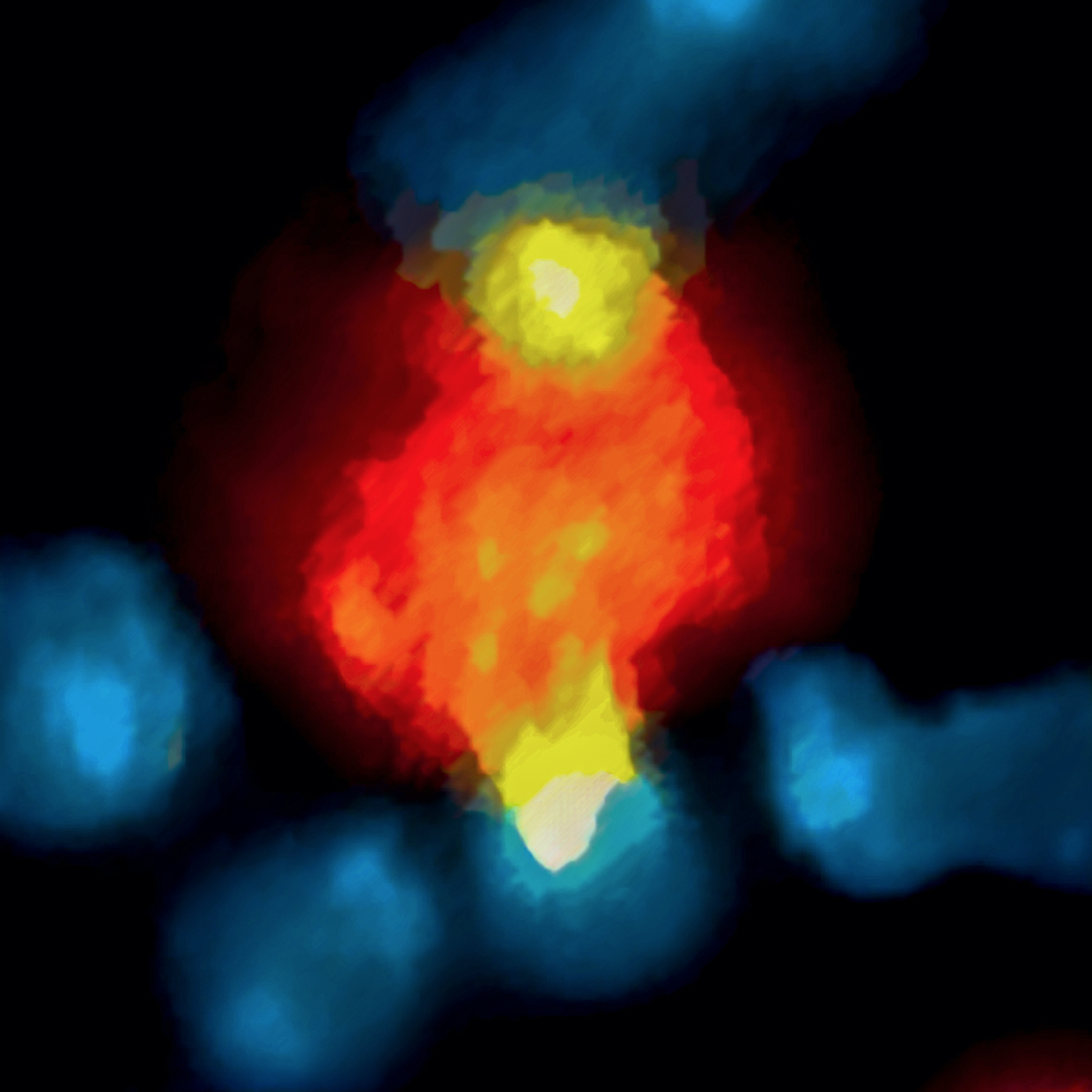
Among today’s most promising weapons against cancer is the use of therapies that direct the immune system against a tumor. One approach—immune checkpoint blockade—is designed to circumvent the “off switches” that prevent the immune system from attacking healthy tissues but can also shield a tumor from the immune response.
These drugs have had remarkable success in some, but not all, patients, and long-term survival has been achieved only in a minority of patients.
Now a study from Harvard Medical School investigators at Massachusetts General Hospital has identified a surprising mechanism for resistance to immune checkpoint blockade.
In their paper published online in Science Translational Medicine, the researchers describe finding that an antibody-based drug designed to block the immunosuppressive molecule PD-1 is removed from its target T cells by macrophages within minutes of administration in several mouse models of cancer.
They also identify the molecular mechanism behind this drug capture, which could lead to ways to prevent the process.
“Immune checkpoint blockers are very effective in some patients but not others, and our current ability to understand why treatments work or fail is quite limited,” said Mikael Pittet, HMS associate professor of radiology at Mass General. “Using high-resolution molecular imaging to track immune checkpoint drugs in real time, we were able to discover what was happening, devise ways to extend the time the drug binds to its target and improve treatment efficacy in our models.”
Immune checkpoint molecules like PD-1 are expressed on the surface of CD8 T cells—the immune system’s “killer cells” that attack damaged or diseased cells, including cancer cells—and act to suppress an inappropriate T cell response. Monoclonal antibodies that block pathways controlled by checkpoint molecules are the basis of current checkpoint blockade drugs.
The research team used intravital microscopy—which examines biological processes in living animals through tiny implanted windows—to track the activity of an antiPD-1 drug in mouse models of colon cancer.
As expected, the labeled antibody was observed to bind to PD-1 molecules on CD8 T cells within a few minutes. But as little as 20 minutes later, the drug had been taken up by macrophages within the tumors. The same process of rapid antibody binding to PD-1 molecules on CD8 T cells, followed by macrophage uptake, was observed in models of melanoma and lung cancer.
To determine how the antibodies were being removed from T cells, the researchers first confirmed that the macrophages neither expressed PD-1 molecules nor take up antibody not bound to T cells.
Experiments in mouse and human tumor cells revealed that antibody removal was accomplished through the interaction of the Fc region—the portion of an antibody that communicates with and directs the action of immune cells—and Fc receptors on the surface of macrophages. Administering an Fc receptor inhibitor prior to anti-PD-1 treatment both extended the binding of the drug to CD8 T cells and led to complete tumor disappearance in a mouse model.
Whether a similar strategy could improve the results of immune checkpoint blockade in human patients may be answered by current clinical trials that combine immune checkpoint blockers with drugs targeting macrophages, which have a number of detrimental effects in cancer.
“Our observations would not have been possible without a method of dynamically imaging drug action on a cellular level,” Pittet said. “Our platform for imaging anti-PD-1 in live animals can easily be adapted to study additional checkpoint blockade agents, so we are building a program to track the cellular interactions that will allow us to decipher drug mechanisms and hopefully leverage knowledge into engineering better therapeutics.”
The study was supported by the Samana Cay MGH Research Scholar Fund, National Institutes of Health grants R01AI084880, R01CA164448, R21CA190344, U54-CA151884, P50CA086355, DP2AR068272-01 and HL084312; Department of Defense grant PC140318, and the David H. Koch–Prostate Cancer Foundation Award in Nanotherapeutics.
Adapted from a Mass General news release.