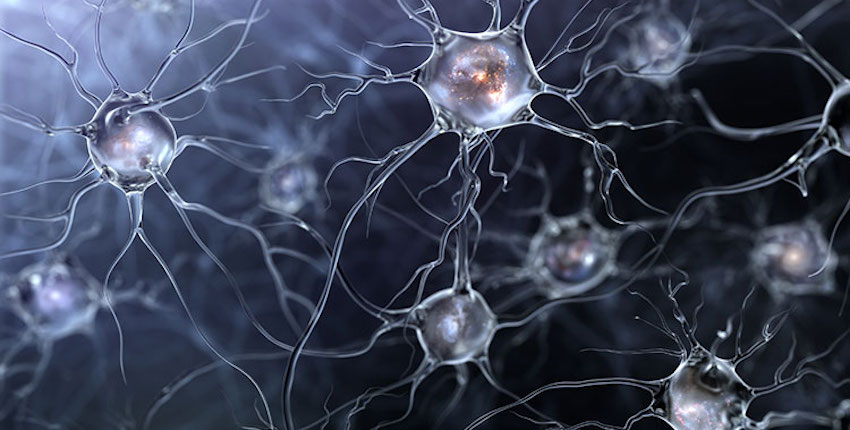
Photo: iStock
Accurate and timely cell-to-cell communication ensures the integrity and normal function of virtually all organisms. Cells communicate with each other by sending chemical “messages,” a process known as secretion, controlled through a symphony of proteins and lipids working together.
While scientists have gained a relatively good understanding of the roles many proteins play in this process, whether and how they interact with key lipids to control cell secretion has remained largely unknown.
A new study, published April 18 in Neuron, by a team led by Harvard Medical School scientists unravels the inner workings of the relationship between one protein-lipid pair in nerve cells, or neurons.
The findings help solve the long-standing mystery of how secretion happens efficiently and at just the right place to ensure optimal cellular communication and could eventually help elucidate what might go wrong in neurologic and other diseases intimately tied to neuronal secretion, including schizophrenia and autism.
“Cell-to-cell communication—be it between neurons or between other types of cells—is critical to ensuring the integrity of multiple physiological functions, such as cognition, muscle movement and many more,” explained study senior author Pascal Kaeser, assistant professor of neurobiology at Harvard Medical School.
“We believe our findings shed much-needed new light on how this process occurs and can help explain what goes awry in a range of diseases,” he said.
Neuronal secretion begins when nerve cells receive a “fire away” signal, a phenomenon known as action potential. When this occurs, lipid-packed bags called vesicles fuse internally with the cell membrane at specialized docking sites known as active zones, located at neuronal synapses, the junctions that connect one neuron to another.
When a neuron gets this “fire away” signal it secretes chemicals within a thousandth of a second, enabling neurons to send messages that induce various sensations or actions, such as pain or muscle movement.
For this to occur, scientists have long known that three things need to occur in perfect synchrony: First, vesicles need to undergo maturation steps, known as docking and priming, that prepare them for rapid fusion. Second, the channels that ferry calcium ions need to be anchored at the docking site. Third, a lipid known as PIP2 must also be present there.
A protein called RIM ensures the first two. However, because PIP2 is present in just a tiny section of the cell membrane, it’s been unclear how it manages to be near the docking site just when it’s needed, especially given the short time scale of vesicle fusion after an action potential.
Suspecting that RIM may also be important for connecting the active zone to PIP2, Kaeser, along with Arthur de Jong, a former postdoctoral fellow in Kaeser’s lab, and their colleagues, investigated whether the pair, in fact, interacts. The researchers examined the sequence of amino acids that make up RIM to see which area might bind to this lipid. Their search landed on a stretch known as the C2B domain.
To test whether this domain is important for secretion, the scientists recorded signal transmission from mouse neurons showing that, indeed, cell-to-cell communication was predicated on a proper connection between RIM C2B and PIP2. This binding didn’t need the influx of calcium from an action potential to take place, suggesting that this protein-lipid pair could be “preassembled” in the cell membrane—a step that might enhance dramatically vesicle fusion after an action potential.
When they removed the C2B domain from RIM in neurons growing in lab dishes, these cells were still capable of releasing some vesicles after the “fire” signal, or action potential. However, the process was extremely inefficient compared to cells with unmodified RIM.
Additional experiments in neurons missing the C2B domain revealed that modified RIM was still present in the active zone and still able to perform its functions for vesicle maturation and anchoring calcium channels, even if it couldn’t bind PIP2 and trigger vesicles to fuse. However, adding just the C2B domain back into cells didn’t rescue this function—a finding that shows that in order for C2B to work, it must be attached to RIM.
Taken together, Kaeser says, these findings closely tie the function of RIM and its C2B domain with that of PIP2. Given that C2 and RIP2 are present in all known cells that have a secretory function, including those that exude hormones, digestive enzymes, insulin and more. Their interaction, the researchers said, likely represents part of a universal underlying mechanism that allows cells to efficiently release signaling chemicals.
“We think this could be a fundamental principle through which cells connect the protein machinery for fusion with the lipid machinery for fusion,” Kaeser says.
“The more we learn about this process, the better we’ll be able to identify the role it plays in a range of disorders.”
This work was funded by grants from the National Institutes of Health (R01NS083898, R01MH113349 and R35NS097333), Lefler Foundation, NWO (Rubicon Fellowship 825.12.028), Harvard Brain Science Initiative and Welch Foundation. Some of the equipment used for the research presented here was purchased with shared instrumentation grants from the NIH (S10OD018027 and S10RR026461). Some of the work was performed at the HMS Neurobiology Imaging Facility (supported by Core Center grant P30NS072030).