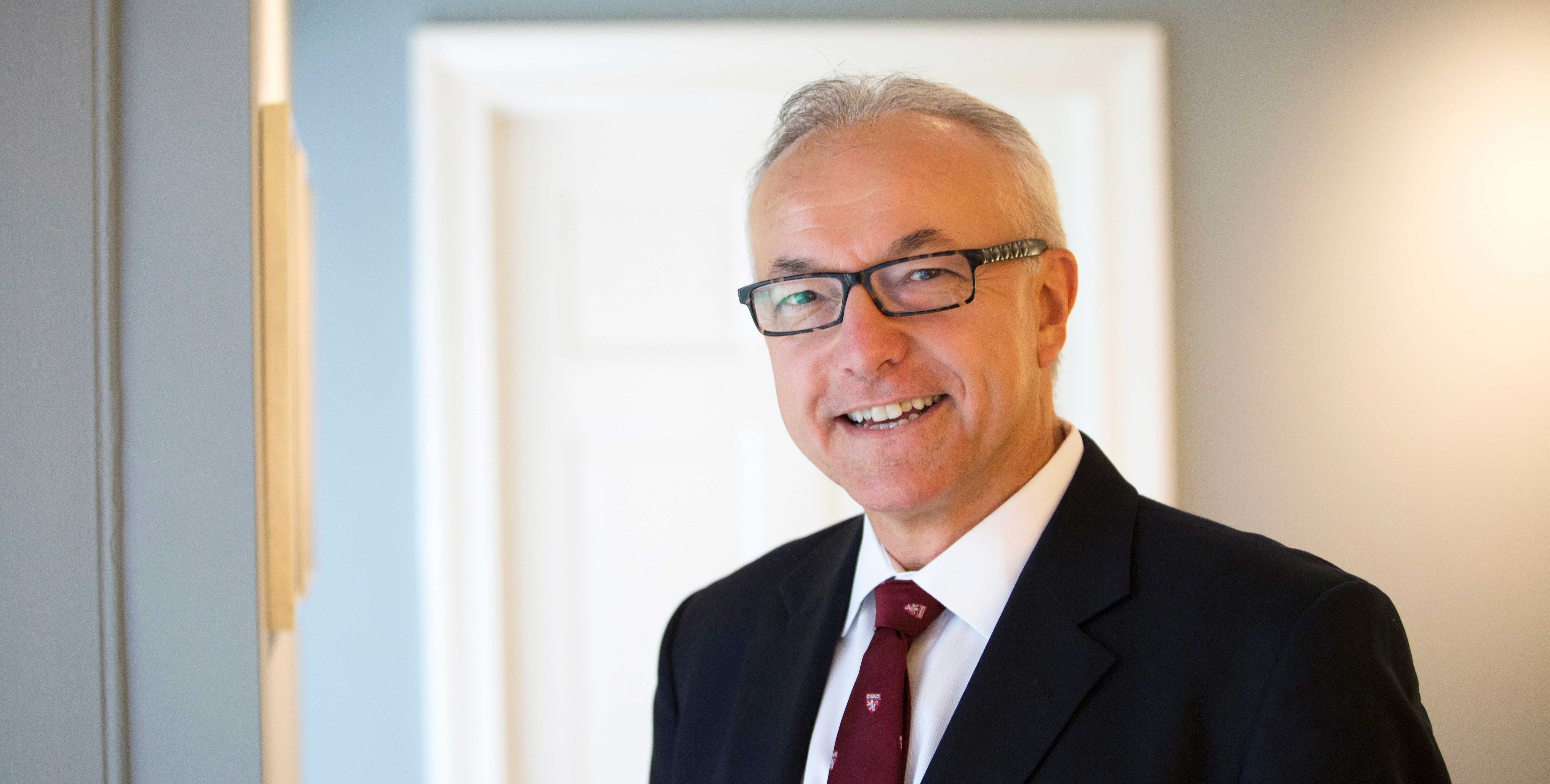
At Harvard Medical School, we sit a mere block away from the building where the legendary Sidney Farber conducted his groundbreaking work that laid the foundation for modern-day chemotherapy.
I think about the late 1940s when Farber was experimenting with aminopterin—a folic acid antagonist—to treat children with acute lymphoblastic leukemia, and then I think about today.
Much of what we are doing now—immunotherapy, cell therapy, gene therapy—would have been deemed science fiction in Farber’s day.
Imagine where we could be 50 years from now.
The past two decades have redefined our understanding of cancer and transformed our ability to treat it. I have personally witnessed tremendous progress in the work of colleagues across Harvard and beyond. I have been lucky enough to experience it in my own lab.
This is truly the century of biomedicine, and I believe cancer is one of the maladies that will experience the greatest transformation as a result of the work that is taking place here in Boston and around the world.
Yet, a few weeks ago, I lost a friend to glioblastoma. It was a painful reminder how much farther we still have to go despite all the progress we’ve made.
At Harvard Medical School and across our hospital affiliates, there are ways I believe we can optimize our successes and clear some of the hurdles that lie ahead.
I believe the most pressing challenges fall broadly into the following categories: cancer biology, therapeutic optimization, forecasting treatment response, individualized risk prediction and access to care.
To solve these challenges, we must focus our efforts on several fronts:
- We must ensure ongoing cross-fertilization, not merely across different areas of expertise, but also across different modes of intellectual inquiry.
- We must get better at propelling basic discovery from bench to bedside, to ensure that fundamental biological insights become clinically relevant treatments.
- We must understand cancer at the single-cell level and within the ecosystem of a whole organism and map out the dynamic relationships between tumor and host.
- We must democratize access to care and lessen the financial toxicity of new treatments.
How do we get there?
Uniquely positioned
I think HMS and the biomedical community of Greater Boston together are uniquely positioned to take on these challenges.
Within a 10-mile radius of this building, there is more scientific talent than arguably anywhere else in the world.
This exhilarating work is happening across biotech and pharma, at MIT and the Broad and, of course, at Harvard Medical School and our teaching hospitals, notably the Dana-Farber/Harvard Cancer Center.
Established in 1998, the Dana-Farber Harvard Cancer Center is the largest cancer consortium in the country, with Harvard Medical School as the nucleus of a system that includes the Dana-Farber Cancer Institute, Massachusetts General Hospital, Boston Children’s Hospital, Brigham and Women’s Hospital, Beth Israel Deaconess Medical Center and the Harvard T. H. Chan School of Public Health.
I believe the most valuable feature of our cancer consortium is its architecture. Built as a matrix, it creates nodes of interaction across various specialties, different scientific disciplines and different modes of scientific inquiry.
These nodes fuel scientific interactivity, they stimulate connectivity, and, perhaps more importantly, open new opportunities for therapeutic translation.
The fruits of these synergies are evident in the number of therapies emanating from them.
Since 2011, the Dana-Farber/Harvard Cancer Center has played a critical role in the development of 45 of 114 FDA-approved cancer therapies.
In 2017 and 2018, the FDA issued 29 approvals for cancer therapies. Clinical trials conducted under our consortium were instrumental for 14 of the 29.
Last year, the consortium had more than 1,100 clinical trial protocols and 14,000 patients enrolled in trials, with nearly 4,300 of them taking part in treatment and intervention trials.
Challenges ahead
These are truly remarkable successes. But we still have a ways to go, and the challenges we face are not unique to Harvard Medical School.
- On the cancer biology front: We have made tremendous progress in understanding cancer at the cellular and molecular levels, but we still face a significant body of “dark matter” in the cancer genome that we need to illuminate.
- On the therapeutic front: we must improve the efficacy of existing therapies and develop new ones. We must develop new synergistic combinations.
- We must get better at predicting how a patient will respond to a given treatment and individualize therapy accordingly.
- We must identify early predictors of both cancer development and of cancer progression.
These are achievable goals and they represent opportunities to turn many cancers into manageable chronic conditions, conditions that patients live with, rather than die from.
Solving these challenges may even cure some forms of cancer. We’ve already seen a sneak preview of this.
In the past 10 or 15 years, we have witnessed two cancer revolutions—precision medicine and immunotherapy, which I’ve had the good fortune to both witness and experience firsthand.
I was personally involved in the research that led to the development of imatinib, better known as Gleevec, in 2001. It is as close to a “magic bullet” therapy as we have for chronic myeloid leukemia, which, until then, was an invariably fatal disease in the absence of a bone marrow transplant.
I’ve had the privilege of witnessing other advances in the work of colleagues and friends.
One such example is the development of smart drugs targeting EGFR receptors to stem tumor growth in certain forms of lung cancer. The basic, translational and clinical research was done right here, at Harvard Medical School, and this work stands as a striking example of precision medicine and a truly field-defining development.
I have seen up close the milestones that paved the way to checkpoint blockade immunotherapy in the work of colleagues, whose labs are at HMS.
Some of the pivotal discoveries into cancer’s ability to evade immune surveillance were made by HMS professors Gordon Freeman and Arlene Sharpe, together with Tasuku Honjo and Jim Allison, among others.
As we all know, these discoveries culminated in the birth of checkpoint blockade therapy, which has redefined the treatment of many cancers.
Immunotherapy is now considered a pillar of cancer care, much like surgery, radiation, chemotherapy and targeted oncogene pathway inhibition.
But we must remember that immunotherapy is still in its infancy.
For every therapeutic triumph there are still many failures, because many patients simply do not respond or go on to develop resistance.
Predicting response, overcoming resistance, lessening toxicity and reducing treatment costs remain serious hurdles for immuno-oncology.
Surmounting hurdles
We are well on our way to surmounting these hurdles. Our ability to profile individual patients’ cancers has grown dramatically as a result of advances in genomics science and sequencing technology. It is now more or less standard of care to sequence the genome of each patient’s tumor to guide therapy.
Case in point: Our ONCOPANEL platform, available to profile patients with cancer in our network. The panel detects mutations in 447 genes, yet this is an evolving field, and we are on the cusp of making an enhancement to this platform as a result of advances in machine learning made by scientists in our Department of Biomedical Informatics.
A team led by HMS's Peter Park just published a new algorithm that can detect with great precision the presence of HR-deficient cancer cells.
The algorithm can be readily incorporated into gene panels currently in use. Doing so would significantly expand the number of cancer patients identified to have HR deficiency who could benefit from treatment with PARP inhibitors that target this DNA-repair defect.
This is just one example of the promise of computational biology and its potential to improve our ability to profile and precision-target tumors so that we can individualize treatments accordingly.
Ultimately, therapeutic success depends on a strong foundation built on basic science, and there is still much we do not know.
To better understand how each cancer arises, we must explore the role that noncoding sequences play in cancer tumorigenesis. Oncogenic mutations can occur in genetic elements beyond the coding region. They can occur in the enhancer, silencer, insulator and promoter sections of the genome.
Illuminating this proverbial “dark matter” can dramatically reshape the way we understand, prevent, detect and treat cancer and I believe these insights will increasingly flow from AI-powered genomic studies.
We remain sub-optimally equipped in our ability to predict treatment response.
For example, we already know that a tumor’s mutational landscape—the number of genetic mutations it contains—can predict response to PD-1 inhibitors. This is likely because their greater genetic diversity makes these tumors more “visible” to immune cells, however, this this is true only in a handful of cancers.
Core principles
What I really look forward to is not merely a compendium of biomarkers, but a set of core principles based on understanding cancer biology at its most fundamental level.
I look forward to a day when oncologists routinely test how a patient’s tumor might respond to five, 10 or 15 agents in the same way we now test antibiotic sensitivity in bacterial isolates.
My colleague, David Weinstock at Dana-Farber, in collaboration with Novartis, has developed what is probably the world’s most comprehensive open-source databank of patient-derived xenograft models, representing more than 750 human cancers. These models can be used to develop biomarkers of tumor vulnerability to specific drugs as well as to explore how and why tumors become resistant to certain agents.
Liquid biopsies will also play an increasingly important role in gauging treatment response as well as in monitoring disease progression. The utility of circulating tumor DNA is already well-established in adults with cancer.
Investigators from Boston Children’s are now working to adapt this technique for use in pediatric patients. HMS scientists have found ctDNA in five of the most common pediatric solid tumors. And their research indicates that the presence of ctDNA correlates with cancer progression in Ewing sarcoma and osteosarcoma.
Liquid biopsies have the potential to help inform clinical decisions regarding treatment modifications. They can identify early non-responders who might benefit from alternative protocols or higher-intensity regimens. Alternatively, liquid biopsies could identify good responders who can be assigned to lower-intensity protocols with lessened short-term and long-term toxicity the latter being particularly important in the pediatric population.
Therapeutic resistance is a pernicious problem that can arise either from primary insensitivity of the tumor or from acquired genetic mutations that eventually render the tumor impervious to treatment. We have identified clues that explain some patients’ lack of therapeutic response to immunotherapy, but the biological mechanisms that fuel primary and acquired resistance remain unclear.
Single-cell sequencing
In this realm, single-cell sequencing will continue to play an increasingly important role. This approach, developed by HMS scientists, enables us to profile individual cells and cell subpopulations, which harbor molecular traits that allow them to escape therapeutic assault and thus give rise to resistance.
An even darker side of resistance is hyper-progressive disease. Some reports suggest that between 9 and 29 percent of patients experience rapid progression, or hyper-progression, following immunotherapy—a disheartening notion. Thus it is critical that we identify genetic variants and other predictors associated with hyper-progressive disease.
The extraordinary complexity of resistance mandates extraordinary collaboration. No single laboratory, no single biologic discipline can conquer it in isolation.
So, under our Ludwig Cancer Center, we have brought together investigators from orthogonal perspectives to tackle resistance on multiple fronts.
To crack resistance, we must understand the tumor-intrinsic and tumor-extrinsic factors that drive it. The tumor microenvironment is a particular challenge Scientists in our Laboratory of Systems Pharmacology recently developed a Google maps-style imaging tool that enables unique visualization of the tumor microenvironment.
This high-throughput, multi-dimensional staining technique reveals the spatial and molecular dynamics between host and tumor cells in fixed pathology samples. Remarkably, it allows us to visually monitor up to 60 different biomarkers at a time on the tissue. In addition to revealing new insights on the tumor microenvironment, one can easily foresee how this technique could be adapted to study drug response.
We can also use tools like this to continue to explore and define the synergistic profiles of combination treatments—old and new alike—to tailor them to individual patients. This depends on a constellation of factors, including timing and sequence. Empirical approaches are important for identifying these combinations, as are studies that shed light on the molecular pathways underlying such effects.
Machine learning
An understanding of the basic mechanisms of these interactions will be critical, of course, but just as important will be computation and machine learning to help us rapidly assess the viability of multiple combinations.
In other words, we ought to move away from bet-hedging, as a recent study from our systems biology department showed, and toward rationally based choices of treatment combinations. Computational models of combinations can identify truly synergistic drug pairings and improve the design of combination therapies.
Reshaping the tumor microenvironment is a critical therapeutic frontier. For example, we now have solid indicators that tried-and-true drugs like losartan, for example, can optimize the effects of chemotherapy in ovarian and pancreatic cancer by inducing changes in tumor microenvironment that weaken the cancer.
We now know that of the myriad cell types present in a tumor, myeloid cells in particular can alter the function of T-cells. HMS researchers like Jennifer Guirrero at Dana-Farber are leading efforts to target tumor myeloid cells, either to eliminate them or convert them to an immune-stimulatory state. This may help optimize responses to both chemotherapy and immunotherapy in drug-resistant breast cancer.
We must remind ourselves that we are in the dawn of immuno-oncology, and our arsenal of immune checkpoint blockade therapies will expand as we understand and harness new pathways.
Gordon Freeman’s lab is currently investigating a novel HHLA2 immune-checkpoint pathway and has identified both stimulatory and inhibitory receptors. This pathway and its receptors are members of the same gene family as PD-1 and CTLA-4.
HHLA2 is over-expressed on a number of tumor types and here is the truly intriguing part: HHLA2 and PD-L1 are generally not co-expressed on the same tumor, raising the possibility that HHLA2 is an alternative, non-redundant mechanism of immune evasion from PD-L1. Gordon and his team have developed antibodies that preferentially block the inhibitory receptor and are exploring their capacity to enhance anti-tumor immunity.
To optimize the effect of immunotherapy, we must understand in greater detail the dynamics of T-cell function and dysfunction. Doing so will give us a clearer understanding of just how immune therapy reinvigorates such dysfunctional immune cells.
These efforts are led by Arlene Sharpe, chair of our newly founded Department of Immunobiology, who recently identified two subsets of exhausted tumor-infiltrating T cells and demonstrated that PD-1 therapy acts on only one of these subpopulations. These cells respond better to PD-1 treatment and can regain their anti-tumor properties. Approaches to expand the population of this subpopulation of T cells might be an important component of improving the response to checkpoint blockade.
Checkpoint blockade, of course, is but one form of immunotherapy, and we must continue to look for other ways to modulate the host immune response. CAR-T therapy and tumor vaccines are the current frontrunners in such next-gen immune therapies.
CAR-T has led to dramatic responses in patients with non-Hodgkin lymphoma and acute lymphoblastic leukemia. For these patients, 9 out 10 respond and more than 80 percent achieve durable remission within 3 months of treatment.
However, CAR-T does not seem to achieve sustained response among those with chronic lymphoblastic leukemia. CAR-T seems to be effective against liquid tumors, but not against solid tumors. When it works, it’s a powerful therapy but not a reliable one. How can we optimize CAR-T for a broader range of attack? It is critical that we answer these questions.
For cancer vaccines, one of the central challenges we must solve is to define the tumor segments most easily recognizable by the immune system.
I will mention one illustrative effort in this area: Dana-Farber oncologist Catherine Wu joined forces with Nir Hacohen at Mass General to develop a fully personalized melanoma vaccine designed from a computational analysis of patients’ personal tumor genomes.
The vaccine has already shown great promise in patients with melanoma. And that same neoantigen-targeting vaccine is now showing promise in patients with glioblastoma multiforme, a truly formidable foe of a cancer with a bleak prognosis. Glioblastoma tumors are particularly hard to crack for two reasons. They have few mutational counts—a feature that makes them less visible to immune cells—and they reside in relatively “cold” tumor microenvironments.
Wu and Hacohen published a study this past January in Nature that shows how their vaccine successfully encourages the migration of a multi-epitope neoantigen-specific T cells to the site of the tumor and how it induced increased numbers of tumor-infiltrating T cells.
Cancer vaccine
In a particularly futuristic twist, scientists in our Wyss Institute for Biologically Inspired Engineering are collaborating with researchers from our hospitals to develop an implantable cancer vaccine, made of a biodegradable polymer scaffold containing growth factors and segments of a patient’s tumor.
In terms of risk prediction and early detection, we are making important headway.
The identification of high-risk individuals with genetic predispositions and the detection of incipient cancers is, without doubt, one of the fronts where we have to focus our efforts.
I’d like to mention the work of Sapna Syngal at Dana-Farber. Sapna is part of a multi-institutional Dream Team that is developing a clinical framework to enable the detection and interception of early pancreatic cancer in people at high risk for pancreatic ductal adenocarcinoma. Sapna, as well as others, are also at the forefront of investigating novel body-fluid biomarkers for early detection of colon cancer that would overcome the limitations of current biomarkers, which tend to miss very early carcinogenesis.
Early detection technologies will also play an increasingly important role, such as the work of Gary Tearney at Mass General, a leader in the field of ingestible diagnostic devices. His lab has developed a tethered optomechanically-engineered pill that obtains microscopic images of the GI tract to detect incipient cancers. The capsule is now being tested as a screening tool for colon, stomach and esophageal cancers.
And I believe that some of the greatest returns on investment in terms of risk-prediction and early cancer interception will come from understanding the fundamental mechanisms of mutagenesis.
Understanding the individual ecology of cancer within each patient is the premise of a project called the Network of Enigmatic Exceptional Responders, led by Zak Kohane in our Department of Biomedical Informatics. This study aims to define the characteristics of patients with cancer who become medical outliers and survive dismal odds, defying prognosis.
In classic diagnostics, a physician must ask two fundamental questions: “Why this patient” and “Why now?”
Zak’s project is asking: “Why is this cancer behaving this way in this person but not in the next?”
This project is built on the axiom that every cancer—and every cancer subtype—is a separate disease, but that there may be key underlying commonalities that may point to a shared mechanism of therapeutic response. So, investigators are collecting genome and exome sequencing data, immune-profiling data, microbiota data, surgical and blood samples, metabolic profiles, lifestyle data, zip code and more to identify why some patients are such extreme outliers.
Cost and access
This is just a sampling of the types of studies, research projects and initiatives that I believe will help propel us forward in our collective ability to solve the challenge of cancer.
But we must acknowledge that some, if not many, of our latest and most transformative therapies remain out of reach for cancer patients—either because of price or sheer availability and access. CAR-T therapies, for example, can cost $400,000 and more.
Solving the issue of cost and access will take social and political will and involve forces outside the control of physicians and scientists. However, we can contribute in at least two ways.
First, as clinicians, we have an opportunity and a responsibility to minimize the use of expensive therapies in those who may be non-responders by routinely screening patients for predictive biomarkers that indicate who will respond to treatment.
Second, we must continue to seek ways to re-engineer biology to make therapies easier to manufacture and therefore cheaper.
In conclusion, to face the many daunting challenges of cancer, we must continue to do what we do best … only better.
Fundamental inquiry has always been our core strength at Harvard Medical School, but we know that our community also has a voracious appetite for advancing basic science into life-changing therapies. Part of our social contract as scientists is that our work should yield relevant insights that can help alleviate human disease and suffering.
To this end, we have launched a major Therapeutics Initiative intended to optimize our ecosystem for translational discovery. This Initiative encourages more vigorous interaction internally, across our departments, institutes and hospital affiliates and also externally with partners from industry.
Ours may be an ecosystem that is uniquely positioned to take on the challenge of cancer but to do so, we must take the blueprint of our successes to date and scale them up by strengthening the connective tissue that binds together academia, industry, advocacy organizations and government.
I call upon all to partner with us on this quest: To transform our understanding of cancer and our ability to treat it, so that we can make it curable and, in some cases, even forgotten.