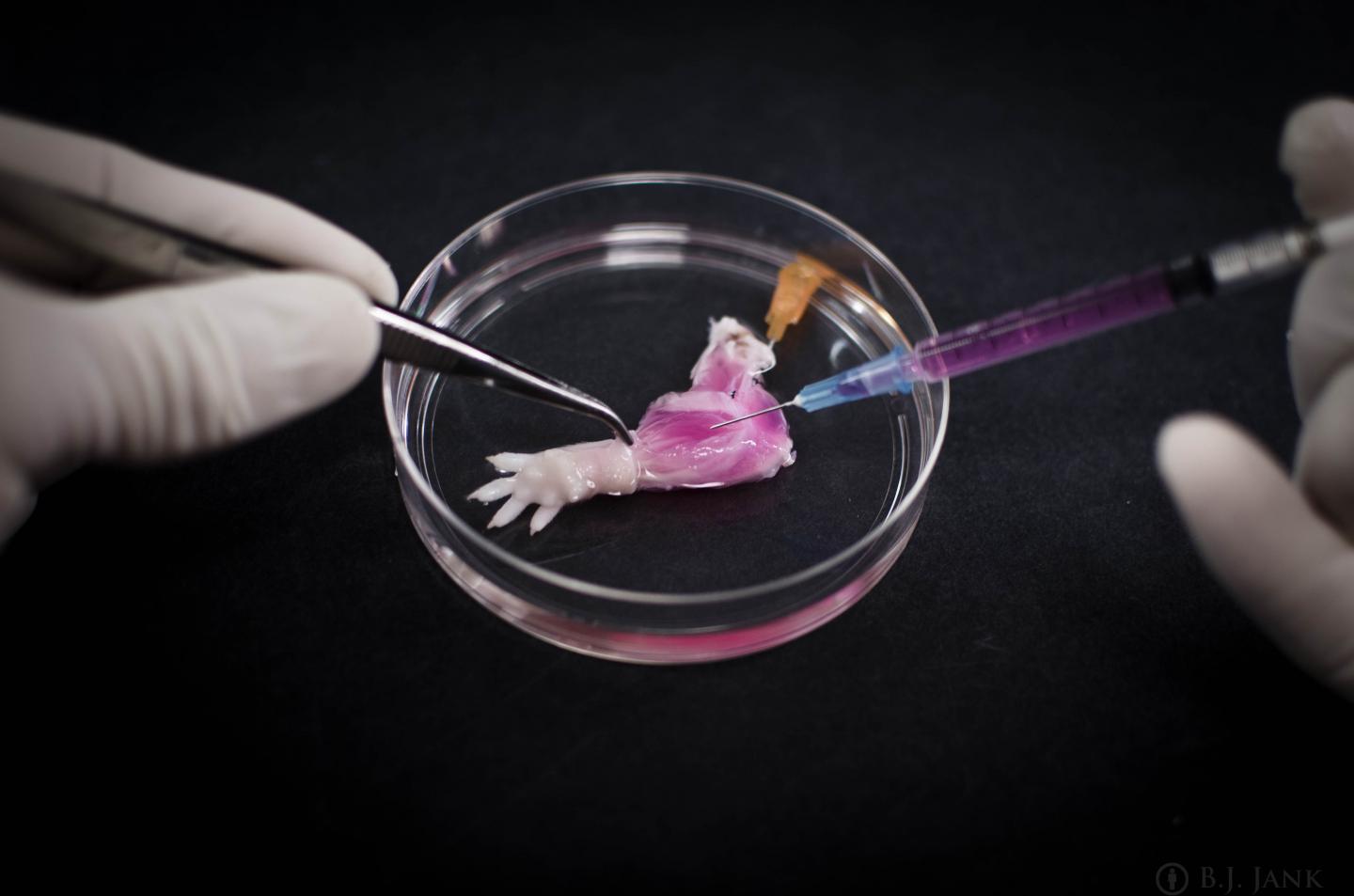
Muscle progenitor cells are injected into the cell-free matrix of a rat limb. Image: Bernhard Jank/Mass General
Harvard Medical School and Massachusetts General Hospital investigators have taken the first steps toward developing bioartificial replacement limbs suitable for transplantation.
In their report, published in the journal Biomaterials, the researchers describe adapting an experimental approach previously used to build bioartificial organs to engineer rat forelimbs with functioning vascular and muscle tissue. They also provided evidence that the same approach could be applied to the limbs of primates.
“The composite nature of our limbs makes building a functional biological replacement particularly challenging,” explained Harald Ott, HMS assistant professor of surgery at Mass General and senior author of the paper.
“Limbs contain muscles, bone, cartilage, blood vessels, tendons, ligaments and nerves—each of which has to be rebuilt and requires a specific supporting structure called the matrix,” Ott said. “We have shown that we can maintain the matrix of all of these tissues in their natural relationships to each other, that we can culture the entire construct over prolonged periods of time and that we can repopulate the vascular system and musculature.”
Harvard Medical School and Massachusetts General Hospital investigators have taken the first steps toward developing bioartificial replacement limbs suitable for transplantation.
More than 1.5 million individuals in the U.S. have lost a limb, the authors noted, and although prosthetic technology has greatly advanced, the devices still have many limitations in terms of both function and appearance. Over the past two decades, a number of patients have received donor hand transplants, and while such procedures can significantly improve quality of life, they also expose recipients to the risks of lifelong immunosuppressive therapy.
Progenitor cells needed to regenerate all of the tissues that make up a limb could be provided by the potential recipient, but what has been missing is the matrix or scaffold on which cells could grow into the appropriate tissues.
The current study uses technology Ott discovered as a research fellow at the University of Minnesota. Living cells are stripped from a donor organ with a detergent solution and the remaining matrix is then repopulated with progenitor cells appropriate to the specific organ.
Ott’s team and others at Mass General and elsewhere have used this decellularization technique to regenerate kidneys, livers, hearts and lungs from animal models, but this is the first reported use to engineer the more complex tissues of a bioartificial limb.
The same decellularization process used in the whole-organ studies—perfusing a detergent solution through the vascular system—was used to strip all cellular materials from forelimbs removed from deceased rats in a way that preserved the primary vasculature and nerve matrix.
After thorough removal of cellular debris—a process that took a week—what remained was the cell-free matrix that provides structure to all of a limb’s composite tissues. At the same time, populations of muscle and vascular cells were being grown in culture.
The research team then cultured the forelimb matrix in a bioreactor, where vascular cells were injected into the limb’s main artery to regenerate veins and arteries. Muscle progenitors were injected directly into the matrix sheaths that define the position of each muscle.
After five days in culture, electrical stimulation was applied to the potential limb graft to further promote muscle formation, and after two weeks, the grafts were removed from the bioreactor. Analysis of the bioartificial limbs confirmed the presence of vascular cells along blood vessel walls and muscle cells aligned into appropriate fibers throughout the muscle matrix.
Functional testing of the isolated limbs showed that electrical stimulation of muscle fibers caused them to contract with 80 percent of the strength that would be seen in newborn animals. The vascular systems of bioengineered forelimbs transplanted into recipient animals quickly filled with blood, which continued to circulate. Electrical stimulation of muscles within transplanted grafts flexed the wrists and digital joints of the animals’ paws.
The research team also successfully decellularized baboon forearms to confirm the feasibility of using this approach on the scale that would be required for human patients.
While regrowing nerves within a limb graft and reintegrating them into a recipient’s nervous system is one of the next challenges facing researchers, Ott said, the experience of patients who have received hand transplants is promising.
“In clinical limb transplantation, nerves do grow back into the graft, enabling both motion and sensation, and we have learned that this process is largely guided by the nerve matrix within the graft,” Ott said. “We hope in future work to show that the same will apply to bioartificial grafts.”
“Additional next steps will be replicating our success in muscle regeneration with human cells and expanding that to other tissue types, such as bone, cartilage and connective tissue,” Ott said.
Bernhard Jank, HMS research fellow in surgery at Mass General, is lead author of the Biomaterials paper.
The study was supported by a New Innovator Award from the National Institutes of Health.
Adapted from a Mass General news release.