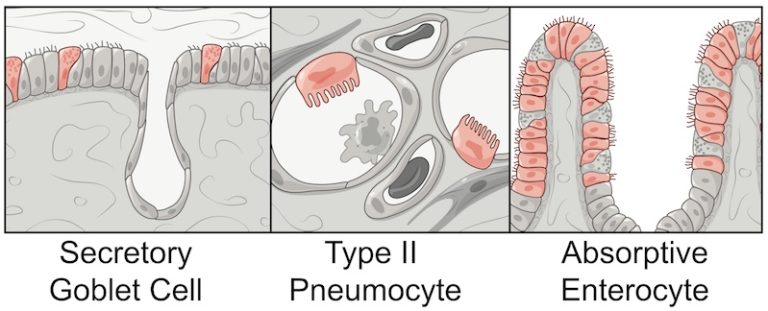
This article is part of Harvard Medical School’s continuing coverage of medicine, biomedical research, medical education and policy related to the SARS-CoV-2 pandemic and the disease COVID-19.
What makes SARS-CoV-2, the virus behind COVID-19, such a threat?
A new study in Cell led by researchers at Harvard Medical School, Boston Children's Hospital and MIT pinpoints the likely cell types the virus infects.
The study also unexpectedly showed that one of the body’s main defenses against viral infections may actually help the virus infect those very cells.
The study, published as a peer-reviewed pre-proof, will help focus efforts to understand what SARS-CoV-2 does in the body, why some people are more susceptible, and how best to search for treatments, the researchers say.
Multiple research models
When news broke about a new coronavirus in China, Jose Ordovas-Montanes, assistant professor of pediatrics at HMS and Boston Children’s, and colleague Alex Shalek at MIT had already been studying different cell types from throughout the human respiratory system and intestine. They also had gathered data from primates and mice.
In February, they began diving into these data.
“We started to look at cells from tissues such as the lining of the nasal cavity, the lungs and gut, based on reported symptoms and where the virus has been detected,” said Ordovas-Montanes, who is co-senior author of the new study along with Shalek. “We wanted to provide the best information possible across our entire spectrum of research models.”
COVID-19-susceptible cells
Recent research had found that SARS-CoV-2, like the closely related SARS-CoV that caused the SARS pandemic, uses a receptor called ACE2 to gain entry into human cells, aided by an enzyme called TMPRSS2.
That led Ordovas-Montanes, Shalek and colleagues to ask a simple question: Which cells in respiratory and intestinal tissue express both ACE2 and TMPRSS2?
To get the answer, the team turned to single-cell RNA sequencing. This identifies which of roughly 20,000 genes are “on” in individual cells.
They found that only a tiny percentage of human respiratory and intestinal cells—often well below 10 percent—make both ACE2 and TMPRSS2.
Those cells fall into three types: goblet cells in the nose that secrete mucus; lung cells known as type II pneumocytes that help maintain the alveoli (the sacs where oxygen is taken in); and one type of so-called enterocytes that line the small intestine and are involved in nutrient absorption.
Sampling from non-human primates showed a similar pattern of susceptible cells.
“Many existing respiratory cell lines may not contain the full mix of cell types, and may miss the types that are relevant,” said Ordovas-Montanes. “Once you understand which cells are infected, you can start to ask, ‘How do these cells work?’ ‘Is there anything within these cells that is critical for the virus’s life cycle?’
"With more refined cellular models, we can perform better screens to find what existing drugs target that biology, providing a stepping stone to go into mice or non-human primates.”
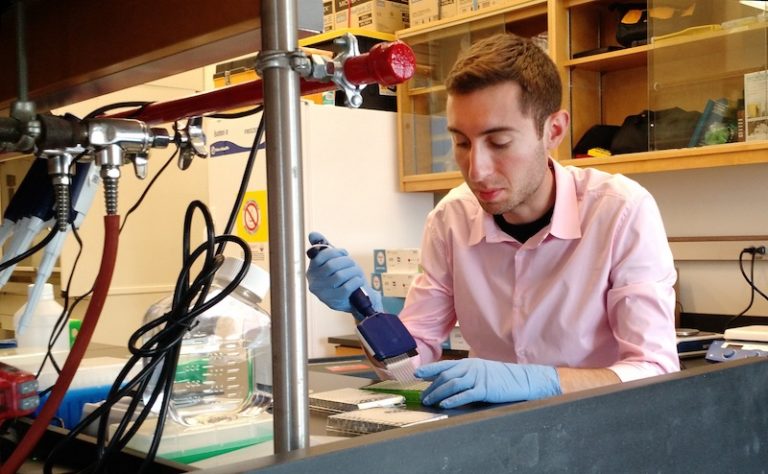
Interferon: Helpful or harmful?
But it was the study’s second finding that most intrigued the scientists.
They discovered that the ACE2 gene, which encodes the receptor SARS-CoV-2 uses to enter human cells, is stimulated by interferon—one of the body’s main defenses when it detects a virus.
Interferon actually turned on the ACE2 gene at higher levels, potentially giving the virus new portals to get in.
“ACE2 is also critical in protecting people during various types of lung injury,” said Ordovas-Montanes. “When ACE2 comes up, that’s usually a productive response. But since the virus uses ACE2 as a target, we speculate that it might be exploiting that normal protective response.”
Interferons, in fact, are being tested as a treatment for COVID-19. Whether they would help or do more harm than good is not yet clear.
“It might be that in some patients, because of the timing or the dose, interferon can contain the virus, while in others, interferon promotes more infection,” said Ordovas-Montanes. “We want to better understand where the balance lies, and how we can maintain a productive antiviral response without producing more target cells for the virus to infect.”
ACE inhibitors and cytokine storms
The findings may also raise new lines of inquiry around ACE inhibitors. These drugs are commonly used to treat hypertension, which has been linked to more severe COVID-19 disease. Are ACE inhibitors affecting people’s risk?
“ACE and ACE2 work in the same pathway, but they actually have different biochemical properties,” Ordovas-Montanes said. “It’s complex biology, but it will be important to understand the impact of ACE inhibitors on people’s physiological response to the virus.”
It’s also too soon to try to relate the study findings to the cytokine storm, a runaway inflammatory response that has been reported in very sick COVID-19 patients.
Cytokines are a family of chemicals that rally the body’s immune responses to fight infections. Interferon is part of the family.
“It might be that we’re seeing a cytokine storm because of a failure of interferon to restrict the virus to begin with, so the lungs start calling for more help," he said. "That’s exactly what we’re trying to understand right now.”
Future directions
In addition, the team wants to explore what SARS-CoV-2 is doing in the cells it targets and to study tissue samples from children and adults to understand why COVID-19 is typically less severe in younger people.
Carly Ziegler, Samuel Allon and Sarah Nyquist of MIT and Harvard and Ian Mbano of the Africa Health Research Institute were co-first authors on the paper. The study was done in collaboration with the Human Cell Atlas Lung Biological Network.
“This has been an incredible community effort — not just within Boston, but also with collaborators around the world who have shared their unpublished data to try and make potentially relevant information available as rapidly as possible,” said Shalek. “It’s inspiring to see how much can be accomplished when everyone comes together to tackle a problem.”
This work was supported in part by the National Institutes of Health (U24AI118672, AI201700104, R56AI139053, R01GM081871, T32GM007753, AI078908, HL111113, HL117945, R37AI052353, R01AI136041, R01HL136209, U19AI095219, U19HL129902, UM1AI126623, U19AI051731, R01HL095791, R33AI116184, U19AI117945, UM1AI126617), Bill and Melinda Gates Foundation, MIT Stem Cell Initiative through Foundation MIT, Aeras Foundation, Damon Runyon Cancer Research Foundation (DRG-2274-16), Richard and Susan Smith Family Foundation, UMass Center for Clinical and Translational Science Project Pilot Program, Office of the Assistant Secretary of Defense for Health Affairs (W81XWH-15-1-0317), P.B. Fondation pour la Recherche Médicale (DEQ20180339158) and Agence Nationale pour la Recherche (ANR-19-CE14-0027).
Adapted from a post in Discoveries, the Boston Children's clinical and research innovation portal.